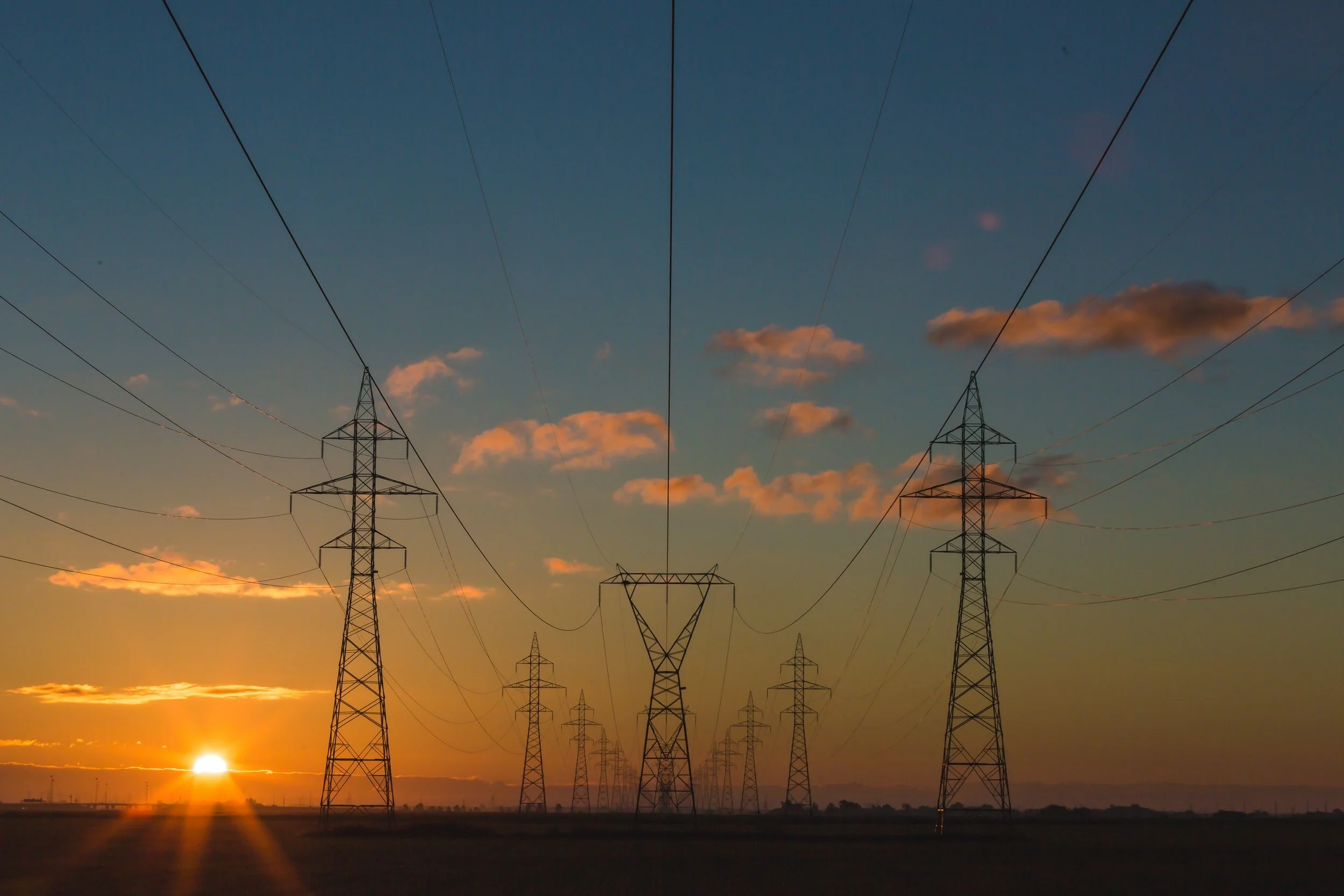
Biophysical Economics
What is Biophysical Economics?
Biophysical economics (BPE) is the study of the ways and means by which socio-economic systems procure and use energy, and other biological and physical non-renewable and renewable resources to produce, distribute, consume and exchange goods and services while generating various wastes and environmental impacts.
BPE examines economic questions grounded in systems thinking and from the perspective of energy and material flows and using the natural sciences as well as the social sciences to understand the socio-ecological implications of humanity’s consumption of resources. BPE is fundamental to understand and inform what could be the sustainable scale of economies. It understands that wealth is derived mostly from the exploitation and transformations of nature using energy, as did many earlier (pre-neoclassical) economists (Hall and Klitgaard, 2018).
BioPhysical concepts influenced the early development of ecological economics by questioning the lack of an energy and material perspective in standard (neoclassical) economics and provided the foundation needed for a paradigm shift in economic thought so that e.g. laws of thermodynamics and conservation of matter were considered and respected (Georgescu-Roegen, 1971, 1993; Odum 1971; Daly, 1968, 1985, 1977; Cleveland et al., 1984; Hall et al., 2001; Melgar and Hall, 2020).
The BPE perspective is essential to address the root causes of ecosystem degradation and unsustainability, which have been primarily driven since the Industrial Revolution by the dependence of economic growth on finite stocks of fossil fuels. This perspective understands that it was only the vast use of low-entropy energy flows from fossil fuels that have provided humanity with unprecedented material well-being (although unequally between and within the global north and south and at the expense of altering the biosphere).
Energy Return on Investment (EROI, or sometimes EROEI)
EROI is a metric that provides a net energy ratio for any given energy gathering activity. Derived by dividing the energy returned to society over the energy invested by society to get that energy (Murphy et al., 2011; Hall, 2011; Hall and Klitgaard, 2018). A commonly used application is a standard EROIstnd that refers to energy directly won from nature, i.e. oil at the wellhead, coal at the mine mouth, corn at the farmgate. Other applications have been derived by expanding the system’s boundary including: point of use EROIpou; extended EROIext; societal EROIsoc; ideal EROIide (Atlason and Unnthorsson, 2014); dynamic EROI (Dale et al., 2012; Capellán-Pérez et al., 2019); and analogous measures such as energy stored and energy saved on investment (ESOI). EROI should always be expressed as a ratio, for example, a mean EROI of 20:1 for wind power means that you get ~20 units of electricity in return (before considering storage costs, e.g. Palmer, 2017) for every unit of energy invested in manufacturing, installing, maintaining and decommissioning a wind energy system (Hall et al., 2014).
Generally, the concept of EROI provides a useful way of thinking about how organisms, ecosystems and societies must obtain enough surplus energy returned from energy gathering activities to live, reproduce and thrive (Hall, 2017; Lambert et al., 2014). EROI is a useful metric to understand and compare the energy trade-offs, efficiencies (and inefficiencies), and surplus potentials of the different fuels and technologies that power, or might power, our socio-economic systems (Melgar and Hall, 2020). While there have been criticisms that EROI estimates are too variable to be useful for analytic work (e.g. Murphy et al, 2016), we believe that paying careful attention to definitions and explicitly stating them greatly reduces the differences in the literature (e.g. Hall and Hanson 2011; Hall et al., 2011; Hall, 2017). As countries embark on an energy transition, it will be vital to understand what is the minimum EROI threshold for society (Hall et al., 2009), and the implications of the large upfront energy investments that will be needed to achieve it (Sers and Victor, 2018; King and van den Bergh, 2018; Capellán-Pérez et al., 2019).
BPE and Energy Transitions
Presently, many societies are attempting an energy transition to low-carbon energy resources due to concerns about depletion, climate change and planetary boundaries (Capellán-Pérez et al., 2014; Steffen et al., 2015). The BPE perspective is critical for addressing and assessing this transition, which will require massive energy and material investments (Capellán-Pérez et al., 2019).
Many biophysical economic thinkers believe that we need to stabilize and reduce (un)economic and population growth to levels that the biosphere can sustain (Daly, 2014). However, they also believe that the lack of a basic understanding of what energy is (the ability to do work) by the public (including decision makers and economists) not only perpetuates a false dualism between humans and nature, but makes it difficult to manage our finite and renewable resources in a sustainable fashion.
Ultimately, BPE aims to teach everyone about the critical role of energy and to help develop policies to examine and analyze the energy flows that power our economies through EROI and other biophysical analyses with the goal of promoting the sustainable consumption of energy and material resources to avoid rapid depletion and the long-term degradation of the biosphere and human suffering.
Would you like to read more? The authors cited above can also be found in these publications.