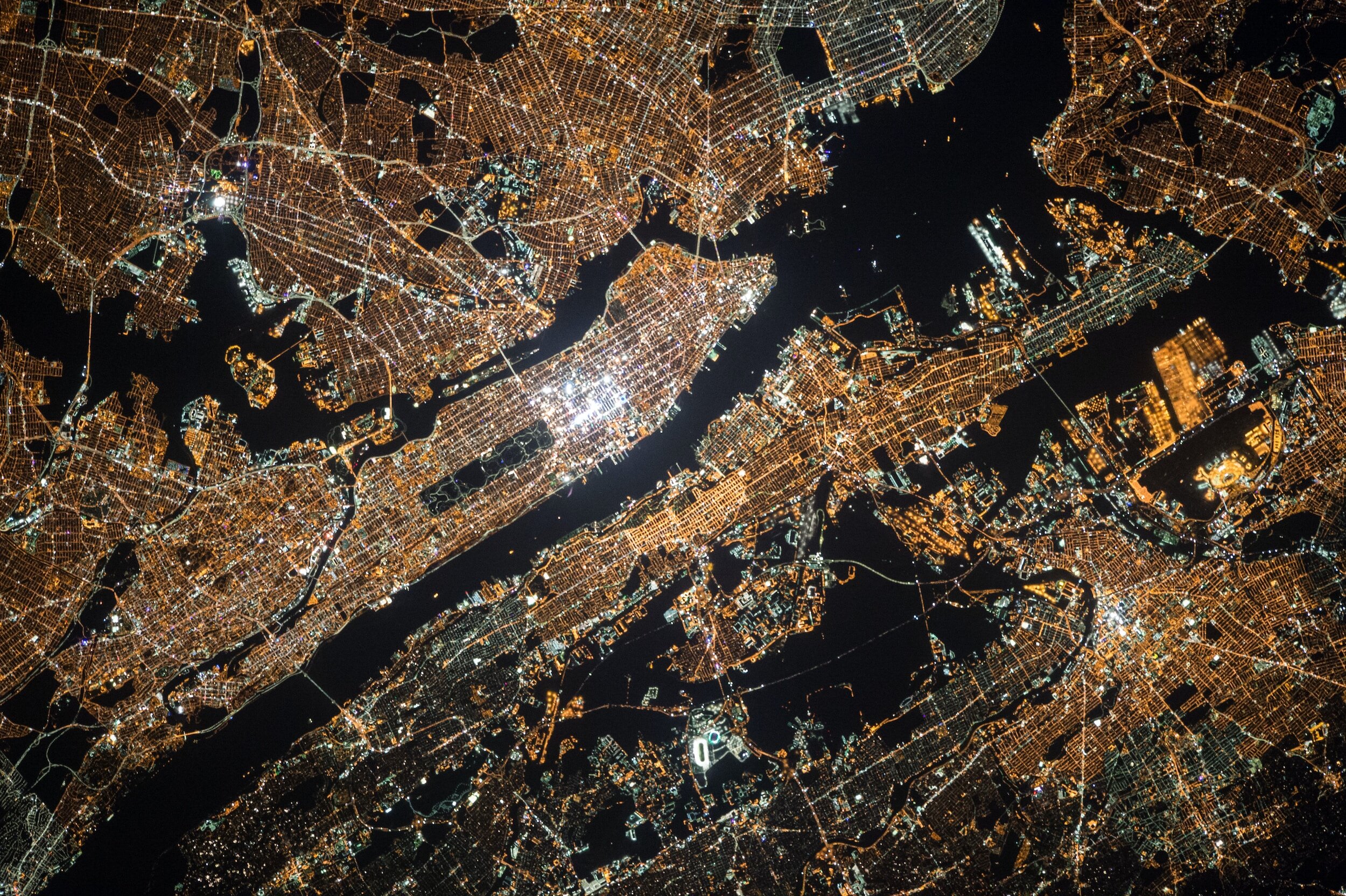
Circular Flow Model & Growth Theory
“Anyone who believes in indefinite growth in anything physical, on a physically finite planet, is either a madman or an economist.”
Neoclassical Circular Flow Model VS. Biophysical Reality
Biophysical and Ecological Economists have pointed out for decades that the circular flow model of firms and households used in neoclassical economics textbooks to represent how economies operate is fundamentally flawed because it violates biological and physical (biophysical) realities. The representation of the economy as an isolated system with circular, self-renewing and self-feeding flows without consideration of energy and material throughputs (inputs and outputs) is considered to be akin to a perpetual motion machine. Below is a visual representation of the standard neoclassical economic circular flow model, and a simple biophysically correct economic model.
Biophysical Critique of the Circular Flow Model
Georgescu-Roegen (1977), Herman Daly (1985), Charles Hall (1986) and many others (Melgar & Hall, 2020) pioneered the biophysical critique of the neoclassical circular flow model based on their understanding that real economies operate within the biophysical world of energy and matter, which is dictated by physical and ecological laws. The physical laws include: (1) The first law of thermodynamics (or the conservation law) states that the total quantity of energy is conserved; and (2) The second law of thermodynamics (or the entropy law) states that the total quality of energy is not conserved. The laws of thermodynamics help us to understand that we can’t make something from nothing and vice versa. The ecological laws include: (1) Transformation of ecosystem structure into economic products degrades and destroys ecosystem services; and (2) waste emissions degrade and destroy ecosystem services. This means that all economic activity requires a source of low-entropy (available) energy inputs to do work (e.g. produce stuff), while generating high-entropy (unavailable or degraded) waste outputs that end up in natural sinks. Below is a visual representation of the laws of thermodynamics.
Economic Growth Requires Available energy
Since the prosperity and stability of modern society is inextricably linked to the production and consumption of fossil energy, amounting for over 80% of total energy consumed, the sustainable quantity and quality of any transition away from fossil fuels should be at the heart of any discussion of economic development. It is obvious that the increase in GDP is closely related to the increase in energy use, implying that energy is required for the increase in wealth to occur. This is consistent with the thermodynamic concept that work (which economic production most certainly is) cannot occur without the expenditure of energy. Below is a more realistic picture of the economic process embedded in society and the biophysical world of energy and matter.
Biophysical Sources
The factors that influence the quality of an energy resource, that is the net energy available to do work for human economies include 1) the quantity of exergy (i.e. energy that can do work, vs anergy or that portion of an energy resource that will be turned into heat) 2) quality of “deposits” (including difficulty in finding and exploiting, size of reservoir, energy density of deposit or source, concentration vs impurities, depth, physical and social difficulty of access, etc.).
Energy surplus is one of the most important biophysical factors because it accounts for the energy availability and quality that flows to the productive economy; i.e., that can allow humans to generate the goods and services they want as well as invest “extra” energy into obtaining more energy to maintain and improve their complex systems. An example of this is provided by King's (2015) study that found that for most of England's history about 40% of all economic activity was used to get the energy needed to run the other 60%. The development of fossil fuels reduced this energy cost to 5–10%. Curiously, economists have argued that since energy's cost share is low it is not important to economies, while biophysical economists argue that au contraire it is the low cost of energy, which has very high economic punch, that makes it so valuable to economies.
Natural Sinks
On the waste side of the economic process, the unavailable energy, or anergy/heat, and the natural sinks that absorb all our waste outputs are also integral components of the socio-economic system. The tendency of increasing entropy as we convert exergy into anergy reduces future energy stocks, especially of nonrenewable energy resources such as fossil fuels. This imposes further biophysical constraints on the ability to perform work and grow the economy. Furthermore, all the waste outputs (e.g., greenhouse gases, plastics, polluted water, etc.), flow into global ecosystems and as emissions exceed absorption rates, they accumulate as noxious stocks.
Neoclassical Growth Theory VS. Biophysical Reality
In neoclassical economics, growth theory, or what is also known as exogenous growth theory, holds that an external macroeconomic factor of technological progress is the main driver of economic growth. Robert Solow (1956) in his “Contribution to the Theory of Economic Growth” developed growth theory through a series of equations based on only using capital and labor as the independent variables, and using the Cobb-Douglas production function to allow his equations to be structured for unlimited substitutable inputs. This allowed his growth theory model to predict steady economic growth and eliminate volatility, while leaving an unexplained 70% “Solow residual” that he attributed to technological progress.
However, Hall and Klitgaard (2018) explain that when Kümmel and colleagues (Kümmel 1982; Kümmel et al. 1985; Kümmel et al., 2002) included energy and creativity (ideas, inventions and values) in the list of independent variables, took the elasticities of the independent variables, and tested them with a LINEX function the residual disappears, with energy being more powerful than labor and capital in driving economic growth.
For decades, biophysical and ecological economists have empathized that not only the real economy is prone to recessions, financial instability, stagnation and even collapse due to biophysical and social limits to growth, but that low-entropy energy is a requirement for any economic process that cannot be ignored by economists (Daly and Farley, 2011; Victor, 2011; Ayres et al., 2013; Daly, 2014; Hall and Klitgaard, 2018).
The standard neoclassical economic circular flow model. Households sell labor and capital to firms, and the firm generates wages and profit in return. The firm produces goods and services by utilizing the factors of production (labor and capital) in exchange for consumption and investment from households. Biophysical inputs of energy and matter and waste outputs are ignored. Source: Hall and Klitgaard (2018)
A simple biophysically correct model of the economy. This ecological economic model represents the reality of the economic system as embedded in the global ecosystems of the world. It includes energy and matter inputs and waste outputs that end up in our global ecosystems. Source: Daly (1977)
A mechanical analogy of thermodynamics. Source: Hall et al. (1986) a graphic representation of the laws of thermodynamics based on an hourglass originally developed by Georgescu-Roegen in 1977.
Raworth (2017) built on the aforementioned thinkers to develop a more realistic picture of how the economy operates embedded in society and the biophysical flows of nature. This economic diagram includes not just the market, but also household production, the government and the commons.
References
Daly, H. 1977. Steady-state economics. San Francisco:W. H. Freeman.
Daly, H.E. and Farley, J., 2011. Ecological economics: principles and applications. Island press.
Daly, H.E., 2014. From uneconomic growth to a steady-state economy. Edward Elgar Publishing.
Hall, C.A. and Klitgaard, K., 2018. Energy and the wealth of nations: An introduction to biophysical economics. Springer.
Kümmel, R., 1982. The impact of energy on industrial growth. Energy 7: 189-203.
Kümmel, R., Wolfgang, S., Alfred, G., and Wolfgang, E., 1985. Technical progress and energy-dependent production functions. Journal of Economics 45: 285-311.
Raworth, K., 2017. Doughnut economics: seven ways to think like a 21st-century economist. Chelsea Green Publishing.